The Interaction Between Light, Molecules, and Nanomaterials to Develop Sensors for Biomedical and other Applications Used to Combat Covid 19
- 30 Sep 2020
- Volume 20
- NANOscientific Magazine, Summer 2020
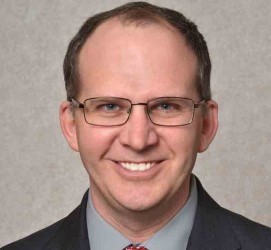
is an associate professor at The Ohio State University. Prof. Schultz earned his B.S. degree from the Ohio State University in 2000 and Ph.D. from the University of Illinois at Urbana-Champaign in 2005. His doctoral studies used infrared-visible sum frequency generation spectroscopy to characterize electrochemical interfaces, work that was recognized with an ACS Division of Analytical Chemistry Graduate Fellowship (2004). Upon completing his Ph.D., he was awarded a National Research Council Postdoctoral Fellowship to conduct research at the National Institute of Standards and Technology (USA) and then as a research fellow at the National Institutes of Health (NIH). His research at NIST and NIH developed vibrational spectroscopy and microscopy methods to study cell membranes. While at the NIH, Dr. Schultz was awarded an NIH Pathway to Independence Award. Dr. Schultz began his independent career as an assistant professor of chemistry and biochemistry at the University of Notre Dame in 2009 and was promoted with tenure to associate professor in 2015. In January of 2018, Prof. Schultz moved his research program to Ohio State. Prof. Schultz was named a Cottrell Scholar in 2013 in recognition of his outstanding teaching and research. Prof. Schultz has served on the Analytical Chemistry Editorial Advisory Board’s Features Panel and is currently on the Editorial Advisory board of Luminescence (Wiley) and Analytical Methods (RSC). In 2019, he was named a Fellow of the American Association for the Advancement of Science (AAAS).
Prof. Schultz’s research focuses on developing innovative approaches utilizing the unique interactions between light and nanostructured materials for near field imaging, ultrasensitive label-free spectroscopic detection, and controlling chemical reactions. His lab studies the interactions between light, molecules, and nanomaterials to develop sensors for biomedical and other applications.
Can you describe metabolites and explain how studying them can help medical research study disease?
Metabolites are the molecules involved in biochemical reactions that control processes in living systems. They include the molecules that are used to synthesize proteins, produce energy and communicate signals in and between cells. The collection of metabolites in an organism is referred to as its metabolome. While proteins are considered the machines in organisms, the concentrations of different metabolites signify which proteins and biological processes are active, or in the case of disease, are being misregulated. Because the body uses many of the same molecules for different functions, the change in a single metabolite is hard to interpret. It is believed that by monitoring as many metabolites as possible at the same time, one gets a better picture of what is happening biologically speaking.
Is there any way studying metabolites helps us understand the COVID-19 virus?
Yes, early on the symptoms and treatment for COVID-19 were based on the activated pathways in the body. Metabolites and other biological markers of inflammation were seen to be causing respiratory distress. There have also been reports of neurological effects, which may result from changes in other biological pathways. Metabolomic studies will play a role in understanding the physiological changes that occur with the disease.
How are metabolites identified and detected?
In metabolomic experiments, the goal is to identify and quantify metabolites. There are more than 40,000 metabolites in the human metabolome. On top that there is evidence that metabolites from the microbiome (bacteria and microorganisms living in our body, like in our digestive tract) also are important in human health. The most common techniques are nuclear magnetic resonance (NMR) and mass spectrometry (MS). MS can detect metabolites at lower concentrations and is probably the most prevalent technique. However, MS experiments typically only detect a few thousand metabolites, perhaps 10% of the known molecules. Additionally, the MS and NMR instruments needed are expensive and found only in shared instrumentation facilities and specialized laboratories. New techniques and methods that can complement existing technologies are active areas of scientific research.
Why is chemical measurement so crucial for scientific breakthroughs. What is your lab doing in this area currently? Please give some examples that your lab is working on.
Being able to identify the molecules in complex systems, such as biofluids or in living cells, typically requires measuring chemical properties. In my lab, we use Raman scattering, or the inelastic scattering of light, to identify and quantify molecules. When a laser is incident on molecules, it causes the molecules to vibrate. The energy transferred into these vibrations changes the frequency (or color) of light that comes off the molecules. By measuring the frequencies of the Raman scattered light, one obtains information about structure and chemical composition of the molecules. The combination of the frequencies and their intensities detected in the Raman scattering can serve as a molecular fingerprint for detection and characterization. Raman spectroscopy is really cool, but the problem is Raman scattering is a pretty weak effect. To make Raman detection work at low concentrations, we use plasmonic nanomaterials to enhance the Raman signal. When molecules are close to, essentially on the surface of, nanostructures; the Raman scattering is significantly enhanced sometimes by more than a billion times. This technique is known as surface enhanced Raman scattering (SERS) [1]. Currently in my lab we are investigating how the Raman enhancements from plasmonic structures and nanoparticles can be used as chemical sensors.
In our metabolomics work, we use the Raman enhancements from a nanostructured silver surface [2]. We have developed a surface that has a high density of nanostructures that generates a significant and reproducible SERS signal. The SERS surface we use was developed in our lab about 10 years ago [3]. We then developed a method to use this surface in an online flow detector to monitor the molecules from biological samples eluting from a chromatographic separation [4]. Our flow detector increases the interactions with the surface to generate improved and quantitative detection. Our most recent publication in this area looked at changes in metabolites with cancer, but we also investigating whether this detector can be used for other samples such as virus particles.
In other projects in our lab, we image the enhancement from nanoparticles to generate Raman maps of samples. Using an atomic force microscope (AFM) with a nanoparticle at the tip apex allows us to collect Raman scattering from the tip apex, known as tip enhanced Raman scattering (TERS), adding chemical information to the measured topography. We have used TERS to monitor protein receptors in intact biological cells to understand chemical interactions related to protein recognition [5]. We have also used gold nanoparticles with multiple branches known as nanostars to study drug targeting in cells [6]. Again, the SERS signal from the nanostars provides chemical information about the chemical environment where the gold nanostar is detected. We are also interested in developing new chemical sensors based on incorporating plasmonic nanoparticles with other materials. Some of these materials can be used for chemically selective or specific detection assays. Others have improved properties arising from the arrangement of the nanostructures and chemical modifications.
How might Atomic Force Microscopes be used to study cells such as in viruses?
Viruses are small, about 50-100 nm in size, and smaller than the diffraction limit of light, which means you can’t see them in an optical microscope. This is a size that is easily imaged using an atomic force microscope (AFM). Viruses were originally visualized using electron microscopes, prior to the invention of the AFM. The AFM has become a powerful tool for visualizing objects in the 1-100 nm size regime. Virus particles on a surface could be imaged by AFM, and differences in virus type may be identifiable by changes in shape. TERS experiments using AFM tips, may be able to further detect chemical differences in the molecules and proteins on the virus surface that may provide targets for therapeutic treatment and diagnostic assays.
How is Atomic Force Microscopy used in the characterization of sensors?
In our lab, we use AFM in a couple ways. The first is in TERS experiments. A nanoparticle on the apex of an AFM tip can be used to increase the Raman scattering from sample, adding chemical discrimination to the surface features measured in AFM. In developing the sensors in our lab, the arrangement, density, and organization of nanoparticles on the surface are important characteristics in sensor performance. We use AFM to measure topography and refine our sensor designs. A real advantage of AFM is the measurement can be done under ambient conditions, or even in solutions, which allows us to characterize our sensors under real life conditions. Changes in the sensor topography are often correlated to changes in performance.
What are the newest discoveries in sensors being developed at your lab?
Our most recent work has been in developing chemically sensitive, but non-specific sensors. What that means is the signal we detect contains chemical information that can identify a specific sample, but many different samples can be investigated. The chemical information in the SERS spectrum arises from the sample, so we have a general-purpose detector. This is important because we can obtain a signal that is specific to a sample without knowing exactly what the sample is. This is incredibly useful when you encounter a new sample or want to detect something that has not been studied before. We are working toward making SERS based sensors quantitative and readily available for identifying new and trace targets in real life samples.
How are virus cells such as COVID-19 viewed under microscope?
As I mentioned in the question about how AFM can be used to study viruses. Virus particles are too small for their shape to be seen in an optical microscope and they require an electron microscope or and AFM to be visualized. Even if the shape cannot be resolved, the Raman scattering from a virus can still be detected in an optical microscope, providing a means to detect their presence without seeing their shape.
What do you think medical science will learn from the current pandemic outbreak?
I think we will learn several things from the current pandemic. Some of the things we may learn have to do with how healthcare is delivered, and outbreaks are monitored. I think one area medical science will advance is in the development of rapid, field deployable sensors. Imagine if every person boarding an airplane could be screened for a virus the way they screen for explosives. Containment and the spread of viruses could be controlled much more readily, preventing an outbreak from reaching the pandemic stage. A real challenge here is detecting something before you know what it is. I hope the sensor development ongoing in my lab can help contribute to this goal.
References:
1. Nguyen Anh, H.; Peters Emily, A.; Schultz Zachary, D., Bioanalytical applications of surface-enhanced Raman spectroscopy: de novo molecular identification. Reviews in Analytical Chemistry 2017, 36 (4), 20160037.
2. Xiao, L.; Wang, C.; Dai, C.; Littlepage, L. E.; Li, J.; Schultz, Z. D., Untargeted Tumor Metabolomics with Liquid Chromatography-SurfaceEnhanced Raman Spectroscopy. Angewandte Chemie 2020, 59 (9), 3439-3443.
3. Asiala, S. M.; Schultz, Z. D., Characterization of hotspots in a highly enhancing SERS substrate. Analyst 2011, 136 (21), 4472-4479.
4. Negri, P.; Jacobs, K. T.; Dada, O. O.; Schultz, Z. D., Ultrasensitive surface-enhanced Raman scattering flow detector using hydrodynamic focusing. Anal Chem 2013, 85 (21), 10159-66.
5. Xiao, L.; Bailey, K. A.; Wang, H.; Schultz, Z. D., Probing Membrane Receptor–Ligand Specificity with Surface- and Tip- Enhanced Raman Scattering. Anal. Chem. 2017, 89 (17), 9091-9099.
6. Sloan-Dennison, S.; Schultz, Z. D., Label-free plasmonic nanostar probes to illuminate in vitro membrane receptor recognition. Chemical Science 2019, 10 (6), 1807-1815.