Critical Emerging Science of Opto electronics Uses Nano-light Probes to Harvest Light
- 01 May 2015
- Volume 4
- NanoScientific Magazine, Spring 2015
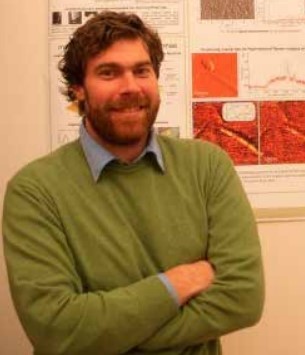
lead Scientist, opto electronics research group at the Molecular Foundry of the Lawrence Berkeley National Laboratory.
Dr. Weber-Bargioni’s research goal is to understand fundamental processes of lightmatter interaction at the nano meter scale with the goal to develop transformative light harvesting and emitting materials. He is leading the opto electronics research group at the Molecular Foundry of the Lawrence Berkeley National Laboratory. He graduated from the University of Konstanz, received his PhD in physics from the University of British Columbia (2007), and did his postdoc at the Lawrence Berkeley National Laboratory. His research group focuses on understanding and controlling fundamental optoelectronic processes at their respective length and time scale, utilizing advancements in plasmonics, near field imaging, and electronic structure and transport studies with molecular scale resolution. For his work he received several awards, such as the DOE Early Career award and the R&D100 award, and teaches at the Technical University Munich.
The Weber-Bargioni group is a highly interdisciplinary and collaborative team at the Molecular Foundry, focused on exploring fundamental optoelectronic nano material properties to ultimately provide a set of rules that enable the systematic development of next generation light harvesting materials. Dr. Weber-Bargioni’s group is focused on imaging and correlating local optical properties and the local electronic structure to provide an insight into optoelectronic processes at the native length scale via state of the art nano optics, Kelvin Probe Microscopy and Scanning Tunneling Microscopy Spectroscopy.
How has nanoscale imaging such as AFM helped you to analyze changes in the electronic structure of light harvesting devices?
AFM is not only good to image the local topography. By functionalizing the tip, e.g. making the tip conductive with a metal layer, we can measure electrostatic forces between sample and tip with high sensitivity or applying bias between sample and tip and measure the local resistivity. Local resistivity, Local Photo Current generation or local Electric Field distributions provide us with insights on the opto electronic processes in light harvesting materials at the length scale these processes happen.
How do nanoscale materials have the potential to create transformative technology?
The fascination of nanoscale materials arises from the fact that material propoertise (e.g. the color, their conductivity, etc) change when we make them smaller then typically 10 nm. Unlike macroscopic building blocks, nanoscale building blocks change their properties also depending on their environment. For example two stones put together to build a house have still the same properties when we put them together. Two nanoparticles attached to each other may change their individual properties substantially. While this is fascinating and provides an enormous amount of possibilities it is also the challenge to understand how do properties change and where can we use it to deliberately design new material properties.
Why is the study of opto electric properties important?
Opto Electronic properties and processes govern an enormous range of applications: Lasers, Solar cells Light Emitting Diodes, Telecommunication, etc... IF we want to either miniaturize devices, or optimize solar cells taking advantage in both cases of the tenability of properties of nano scale materials we need to understand how do these properties change at the nm scale, how are they modified in different configurations and can we use that to make e.g. a solar cell that works at the theoretical limit. TO do so we cannot integrate over many processes of many different nano building blocks, but we need to be able to visualize individual processes in individual nano building blocks to know how to engineer novel materials.
What is a nano light probe and how is it used is optoelectronics?
Nano Light probes are nanofabricated optical antennae. The concept of optical Antennae has been discovered only a few years ago, whereby we use nanofabricated optical antennae to squeeze light well below the previously thought smallest light spot possible, also called the diffraction limit. With that we can optically excite a material with just a 10nm spot and study using optical spectroscopy with a spatial resolution of 10nm the opto electronic properties of a material.
What kind of research have you been doing on new nano materials?
Can you explain what perovskites is and why it is an important discovery? We study opto electronic processes in perovskite materials because they are a new class of thin film PV materials which have a power conversion efficiency of 20% and this was achieved by only a few research groups in barely 5 years - because of the success there are now of course many research groups jumping on this materials such as my group. We hope to map and understand the local process of light to electric energy process conversion and where the bottlenecks are to provide a systematic pathway of optimization of these materials towards the theoretical limit.
What types of advanced imaging techniques do you commonly depend upon for your research to ensure accuracy?
My laboratory is heavily focused on Scanning Probe Microscopy and light. We have a Scanning Tunneling Microscope that works in Ultra High Vacuum with a base pressure lower then what you have in outer space – at least in our solar system It also works at 4 Kelving – so just 4 degrees Celsius above the absolute lowest temperature – and therefore we have the stability to see individual atoms, push them around, and very important for us – we can image the spatial extend of the molecular orbitals of individual molecules. We have a Near Field optical Microscope where we combine Nano Optical Antennae at the end of a scanning probe microscope tip to scan it over the sample and study optically the properties of materials, e.g. how efficient can we excite a material in one position versus another position. Last but not least we use a Park AFM in a controlled environment (glove box) where we can couple light in which mimics the solar spectrum to study how new solar cell materials operate at the lengthscale where optoelectronic processes happen.
“THE MOTIVATION IS SIMPLE: HUMANITY MAKES IPHONES THAT FIT IN OUR BACK POCKET WHILE CONTAINING MORE COMPUTING POWER THEN THE GUIDANCE COMPUTERS FOR THE APOLLO 11 MISSION. WE REALLY SHOULD BE ABLE TO DEVELOP MATERIALS THAT COST EFFECTIVELY CONVERTS THE FREE SUN ENERGY INTO ELECTRICAL AND CHEMICAL ENERGY.”
DR. ALEXANDER WEBER-BARGIONI, LEAD SCIENTIST OPTO ELECTRONICS RESEARCH, MOLECULAR FOUNDRY, LAWRENCE BERKELEY NATIONAL LABORATORY
What can we learn about harvesting light to improve conditions on the Earth that might revolutionize the future of society?
The question is not what we can learn about harvesting, the question needs to be for our society how can we optimize light harvesting and use it as our main energy source! I and many other scientists believe that the most pressing challenge of our time is to come up with sustainable energy sources that guarantee our kids and generations to come to still be able to live on this planet. I am unfortunately already doubtful if future generations will have the quality of life that we in the western society were allowed to enjoy. Unfortunately Humans think short term and hence the only argument that seems to fly is if solar becomes cheaper then other energy sources – regardless of the implication of tomorrow. To do so there is only one way: Enhance the efficiency of solar cell devices. Cheep materials don’t have as much of an impact as on the prize (because installation and overhead cost considerably more then typical cell manufacturing) as enhancing the efficiency. To enhance the efficiency we need to operate as close as possible to the theoretical limit which is 28% for a single junction cell, 47% for a tandem solar cell. To reach these high efficiencies we have to make sure that the solar cell absorbs all light impinging on the cell and especially that every photo charge that is created finds it way to the electrode and can be used. To make sure that all photo charges are used, we need to image the processes at the lengthscale of where these opto electronic processes happen.