Hair Damage From Sunlight Radiation Observed Using AFM: High School Intern Alvin Lee, Monta Vista High School
- 26 Sep 2016
- Volume 8
- NanoScientific Magazine, Fall 2016
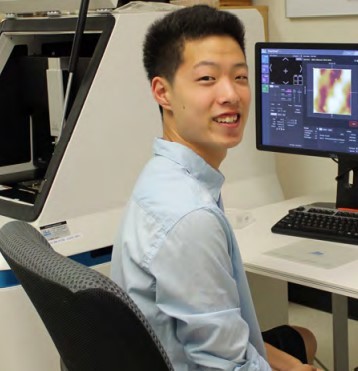
By Alvin J. Lee, Monta Vista High School, Cupertino, California
Abstract
Products for sun protection such as hats, sunscreen, and clothing are commonly used to prevent skin irritation and damage, but few products for protecting hair exist. Through researching the radiation effects of sunlight on hair, it is to be determined whether or not this is a concern worth looking into, especially for those with less photostable, fair-colored hair. With the Park NX20 Atomic Force Microscope (AFM) and its user-friendly Park SmartScan software’s Auto mode, images of hair can be produced to compare its nanoscale topography before and after prolonged exposure to sunlight. The post-exposure sample analysis will be focused on topographical changes indicative of structural damage, as well as a cross section view analysis to assess possible internal changes. Several 5 μm x 5 μm areas on the hair are examined to quantify damage in terms of change in surface roughness value (Rq). Both types of hair were observed to lose surface roughness after prolonged sunlight exposure with black hair decreasing in roughness 77.3% less than blonde hair did. This indicates that prolonged sunlight exposure smoothens hair by wearing out its surface layers evenly and that black hair is more resistant to this process than blonde hair is. This observation is further supported our cross section data as black hair’s cuticle layer width decreased by 0.1 μm and whereas a cuticle layer decrease of 1.5 μm was observed in blonde hair.
Introduction
This study's objective is to observe the effects of long term exposure of sunlight on hair fiber. Many fear the damaging effects of prolonged sunlight exposure on skin, yet few stop to think about the implications of such damage on hair. Black hair is known to be more resistant to sunlight damage than blonde hair [1] due to black hair containing the photo-unstable pigment pheomelanin in levels only a fifth of what is found in blonde hair [2]. This key difference suggests that solutions to mitigate the damage of prolonged sunlight exposure on hair will need to vary based on the target population's hair color. Using a Park NX20 AFM system, the damage done by sunlight radiation on black and blonde hair will be compared by means of comparing surface roughness values (given as Root Mean Square roughness, Rq) before and after receiving a standardized dose of extended sunlight exposure.
Because blonde hair contains five times more pheomelanin (a substance known for its low photostability) than black hair, it is expected that more free radicals will be produced in blonde hair. These free radicals are chemically reactive particles created in the presence of radiation. They effectively destroy the hair cuticle by reacting with nearby stable molecules and eroding the hair's natural structure [3]. Hair after prolonged exposure to sunlight is deduced to be rougher due to what is expected will be an uneven formation of craters and pockets caused by free radical wear. Consequently, changes from this radiation should also be visible in the cross section as we expect that the cuticle layers will thin out.
Experiments
Using the Park NX20 AFM, nanoscale images are able to be produced by tracing the topography of the scan site with a probe. The AFM probe will move up and down based on the topography of the sample and using the captured probe movement data, the Z position at each point on the sample can be translated into an image. The AFM fits the scope of the research for several important reasons. The most commonly used instrument for looking at hair on a very small scale is the scanning electron microscope (SEM). The problem with use of an SEM instrument is its requirement for a conductive surface. This sample preparation is not only tedious and expensive, but it also alters the sample and its composition. Furthermore, the AFM is also able to track many of the sample's mechanical properties such as roughness—one of the key values that will be used in the analysis.
Virgin straight hair from the author and virgin straight blonde hair from a true blonde 24-year old female volunteer are imaged under the Park NX20 to examine their natural topographies. The samples are mounted onto a piece of carbon tape to aid both the accurate landing of a non-contact (such as PPP_NCHR or OMCL-AC160TS) AFM probe tip onto the surface of the hair as well as the increase the stability of the sample as it is scanned. To maintain a consistent tip-sample landing location, landmark features of the area near the hair are observed and selected, and the areas of carbon tape near the hair were pushed in using tweezers under the optical microscope for positional accuracy. All operations of the Park NX20 were facilitated with Park SmartScan, the tool's operation software. Non-Contact mode was used throughout all AFM operations.
To apply a treatment of prolonged sunlight exposure, hair samples, hereafter referred to by their color ("black" and "blonde") are left out in direct sunlight in a petri dish for a total of 42 hours, ranging from 6 to 8 hours of exposure at a time. During the hours of exposure, the UV index is recorded to quantitatively assess the intensity of the sunlight. Over the course of 42 hours, the average UV index was 7.6.
To analyze possible internal damage, black and blonde samples from before and after the sunlight exposure treatment were cross-sectioned. The hairs were laid out, lined up laterally, and embedded in an epoxy to be cured. The cured epoxy is then turned upright so that the tips of the hair pointed upwards (see Figures 4a-d). The top of this setup was then ground down and polished with silica film, lowering the roughness to 0.04 μm.
RESULTS & DISCUSSIONS
Surface Roughness. To compare roughness in Rq, the 5 μm by 5 μm images were flattened once in the X direction and once in the Y direction based on which linear regression fit the peaks the best. This is to avoid any macroscopic contour. These 5 μm by 5 μm regions are found by taking a larger image to get a complete view of the entire hair sample and then choosing a region in which there are no visible steps or scales in the hair (see Figure 1a). For sunlight-treated hair, three 5 μm by 5 μm regions were taken. The bottom 5 μm by 2 μm area of the selected region is then used to find the roughness (see Figure 1a for example). After 42 hours of sunlight radiation, surface roughness decreased in both black and blonde. The images shown are the non-flattened scans displayed with a high-contrast color filter.
Figure 1a. iExample of selecting a 5 μm x 5 μm region on a sample hair for surface roughness analysis. Above is a 40 by 100 μm AFM topography image of black hair after 42 hours of sunlight radiation. A small region on the surface, free of any step or scale edges, has been chosen for roughness measurement.
Figure 1b. 3D AFM topography image of the sample in Figure 1a. Because the AFM is able to process Z-directional data by moving the tip up and down, as well as track from side-to-side, such images as above can be produced. 3D renderings of the AFM topography were invaluable in confirming the selected site for roughness measurement was free of step and scale edges.
Figure 2. AFM topography images of the black hair samples: (a) before any prolonged exposure to sunlight; Rq: 4.5 nm, (b) first sample after 42 hours of sunlight, Rq: 4.1 nm, (c) second sample after 42 hours of sunlight, Rq: 4.9 nm, and (d) third sample after 42 hours of sunlight, Rq: 3.1 nm. The green box in Figure 2a is the region in which the Rq value was calculated for that sample; regions of identical dimensions and position were used to calculate the Rq values of the other samples.
All topography images in Figure 2 have peaks colored orange due to the high-contrast filter. Seen above, the Rq values actually decreased after sunlight exposure occurred, contrary to our hypothesis. The difference of the average untreated hair Rq and the average treated Rq is 0.5 nm. The image of the untreated sample (Figure 2a) contains visible bumps that were most likely eroded from due to free radical damage and flattened out until it became similar to the surfaces in Figures 2b-d.
Figure 3. AFM topography images of the blonde hair samples: (a) before any prolonged exposure to sunlight, Rq: 8.2 nm, (b) first sample after 42 hours of sunlight, Rq: 7.4 nm, (c) second sample after 42 hours of sunlight, Rq: 7.0 nm, and (d) third sample after 42 hours of sunlight, Rq: 4.0 nm. The green box in Figure 3a is the region in which the Rq value was calculated for that sample; regions of identical dimensions and position were used to calculate the Rq values of the other samples.
ΔRq of the untreated blonde hair and of treated blonde hair is, on average, 2.1 nm. This is nearly four times that of black hair. Based solely on the visual qualities of the topography seen in each image of Figure 3, it is evident that the roughness would indeed decrease. While blonde hair does have many bumps on the surface after the 42 hours of irradiation, the pre-treatment sample (Figure 3a) had large peaks and craters on the surface which are not observed in its post-treatment counterparts.
Cross Sections: By examining the width of the cuticle, approximately how much of the hair was worn out after prolonged exposure to sunlight can be determined. Theoretically, because of the radiation-induced free radicals, we should see evidence of damage such as our samples’ cuticles decrease in size. Both topography and amplitude data were recorded when scanning the cross section with AFM. Amplitude imaging was used primarily to help produce high-contrast visuals to make the cuticle region more visible and identifiable for analysis.
Figure 4. AFM topography (left) and amplitude (right) images of cross sectioned hair samples: (a) black before 42 hours of sunlight exposure, cuticle width = 2.1 μm, (b) black after 42 hours of sunlight exposure, cuticle width = 2.0 μm, (c) blonde before 42 hours of sunlight exposure, cuticle width = 2.6 μm, and (d) blonde after 42 hours of sunlight exposure, cuticle width = 1.1 μm. The cuticle width is estimated by measuring the distance between the two triangular bluish color markers shown in the each amplitude images.
According to our cross section results, it appears that both black and blonde cuticles got smaller after the sunlight treatment: black decreased by 0.1 μm while blonde decreased by 1.5 μm. Knowing that blonde is less photostable than black hair and will therefore be more susceptible to sunlight damage, this data supports the idea that increased pheomelanin levels in blonde hair correlate to increased photodamage as compared to their black counterparts.
Conclusion
It appears that because both types of hair had their surface roughness values lowered as a function of time when exposed to sunlight, prolonged exposure to sunlight affects both dark and fair-colored hair. The form of this damage did not come in the hypothesized form of increased roughness however, as both the surface roughness and cross section analysis suggest that sunlight radiation actually smoothens the surface of hair decreasing the width of the cuticle in a more uniform manner than expected. It also appears that the ΔRq for blonde hair is greater than that of black hair despite being exposed for the same amount and intensity of sunlight, This suggests black hair degrades at a slower rate than its blonde counterpart does, an effect that is supported by previous research into pheomelanin levels in these types of hair.
Because the cuticle is the layer meant to receive the most wear, it would make sense that structurally, the hair will become weaker as the cuticle thins out. Studies show that tensile strength (breaking resistance when pulled) decreases after hair has been exposed to certain UV treatments [4). Other mechanical properties that would most likely change due to prolonged exposure to sunlight are ductility and friction. Frictional force would likely decrease due to the roughness of hair decreasing after exposure. It would seem that hardness would not change much due to the cuticle still remaining after exposure, but it may change due to the chemically reactive species (free radicals) making new byproducts that are different in hardness than the proteins that make up hair.
Based on the findings, hair damage is not much of a high priority concern for black haired individuals, as the cuticle only decreased in width by 0.1 μm, a distance almost negligible as it is less than 4% of the original width. Blonde hair, on the other hand, may need much more tending to when under a large amount of exposure. Over the course of 42 hours, the blonde hair cuticle decreased in width by more than half its original width (1.5 μm down from 2.6 μm), meaning in less than 84 hours, there would be no cuticle left to protect the surface of the hair. To confirm, the initial pre-treatment cuticle thickness of our samples are in line with reports using much larger sample sizes [5]. The relationship of pheomelanin to this phenomenon, if any, could not be clearly identified within the scope of this study. With pheomelanin known to be housed in the hair shaft's cortex rather than in the exterior cuticle, any free radical-caused damage would have to travel outward through the hair shaft to affect the cuticle layer. The effect of this speculated free radical travel was not visually detected in either the topography or amplitude images of the post-treatment sample cross sections. It is quite possible that the high levels of cuticle degradation in blonde hair may be due to another unidentified structural factor.
Keeping only the observable cuticle damage in mind, the mechanical properties of blonde hair will have been negatively affected. For example, sun-damaged blonde hair may have also affected the keratin bundles in the shaft's cortex, making the hair much easier to snap due to a decrease in tensile strength. There are, however, products on the market such as keratin-based shampoos that have been promoted as being essential in repairing damage from sunlight. The veracity such claims by existing products were beyond the scope of this study, but may be a fruitful topic to explore in the future.
Finally, a more controlled environment may be better for testing property differences in hair. For example, had a UV lamp been used rather than sunlight so that one could quantitatively assess how much radiation went into the hair, it would be easier to show the changes in hair as a function of time exposed to "x" amount of radiation. Because the UV index was specifically designed for skin damage when it was first created, it may not have been the best form of measurement to assess UV exposure, but other tools for recording sunlight intensity were not available at the time of experimentation. Such considerations would be well placed in follow-up studies on this very topic.
Acknowledgments
Thank you to Park Systems, Dr. Byong Kim, Dr. Mina Hong, Gerald Pascual, and Keibock Lee for making this research possible.
REFERENCES
(1) Lee, W.S. "Photoaggravation of Hair Aging." International Journal of Trichology. Medknow Publications, Dec. 2009. Web. 09 Aug. 2016.
(2) RM, Tyrell. "Result Filters." National Center for Biotechnology Information. U.S. National Library of Medicine, n.d. Web. 10 Aug. 2016.
(3) Monteiro, V.F. "UV Radiation: Aggressive Agent to the Hair--AFM, a New Methodology of Evaluation." Journal of Cosmetic Science (2003): 1-11. Web.
(4) Noguiera, A.C.S. and Joekes, I. "Hair Melanin Content and Photodamage." Journal of Cosmetic Science (2007): 1-7. Web.
(5) Takahashi, T., Hayashi, R., Okamoto, M., and Inoue, S. "Morphology and properties of Asian and Caucasian hair." Journal of Cosmetic Science (2006): 57, 327-338. Web.
Appendix A
The Structure of the Human Hair Shaft
A hand-drawn cross section of a hair shaft from Henry Gray’s Anatomy of the Human Body.
Each strand of hair on your body is composed of layers of structures that each have specific functions. The outermost layer, the cuticle, is the main focus of this study. It is formed from sheets of thin dead cells that overlap one another, producing scale-like structures that can be seen with a microscope. The cuticle provides protection for the interior of the hair’s shaft and is even coated with lipids to help repel water. Contrary to popular belief, the cuticle does not give hair its color; in fact, it is transparent and exposes the next structural layer underneath.
The color of one’s hair is actually determined in this next layer, the cortex. This region is the most highly organized area of the hair shaft containing both the keratin bundles which give hair its mechanical strength as well as melanin pigments. The number, distribution, and types of melanin in the cortex are what determine the hair’s color. Pheomelanin, the substance hypothesized to be the critical factor causing the difference in damage levels in this study’s samples, along with eumelanin are the two types of melanin found in hair. The innermost structure in the hair shaft is the medulla. This region is perhaps the least understood of the entire
The innermost structure in the hair shaft is the medulla. This region is perhaps the least understood of the entire shaft, but can be likened to the pith of a fruit skin or bone marrow. Mitochondrial DNA can be extracted in large amounts from the medulla—a process useful for some types of forensic investigation.
Appendix B
Basic Modes of Operation in AFM
A basic setup for non-contact mode atomic force microscopy. Note the deflected red laser beam (A) shining back up into a sensor (B). This allows the system to keep track of changes in the probe’s (C) position. This vertical information along with recorded lateral movements are then combined by software to render an image of the sample’s topography.
Atomic force microscopes (AFM) are tools that can gather topographical information by running a mechanical probe across the surface of a target sample. This mechanical probe consists of a spring-like cantilever which has a sharp tip fixed to its free end. AFM systems can be run in one of several basic modes of operation:
(1) Contact mode. In this mode, the probe is brought into physical contact with the sample and is gently traced across laterally line-by-line until the entire sample has been scanned. Over the course of the scan, the probe's vertical motion as it rises with peaks, sinks into dips, and twists around features on the sample's surface are recorded. The lateral and vertical movements of probe are combined by software to produce an image of nanoscale features at the scan site.
(2) Non-contact mode. This was the mode used in this hair study. As its name suggests, the probe's cantilever is made to oscillate at a designated reference amplitude just above the sample surface. The probe tip avoids making direct physical contact with the sample as it scans. As the probe tip approaches the sample surface, the amplitude of the cantilever's oscillation decreases. The system's built-in feedback loop detects this change and lifts the probe such that the cantilever oscillation returns to its original reference amplitude. These precise vertical movements are then combined with other recorded lateral movements to render an image of the scanned site.
Along with non-contact mode, another AFM term used in this study was amplitude imaging. An AFM amplitude image is a 2D display of the error signal obtained when using non-contact mode. It shows the degree at which the cantilever's oscillation amplitude deviated from its original reference amplitude at single points in time. This is helpful in visualizing certain topographical features with dramatic slope changes such as steep drops and sharp climbs (places where amplitude can change dramatically in a short amount of time), but is less useful in imaging existing features with more modest, steady changes in slope like shallow depressions (places where amplitude changes take place over large amounts of time).
(3) Tapping mode, is also used by some AFM users. Usually considered an alternative to non-contact mode, this technique also oscillates the cantilever above a sample surface but at much higher amplitudes. While capable of producing usable images, the tip deterioration rate is accelerated—a problem to keep in mind as blunted tips produce lower resolution images and need to be replaced for optimal results.